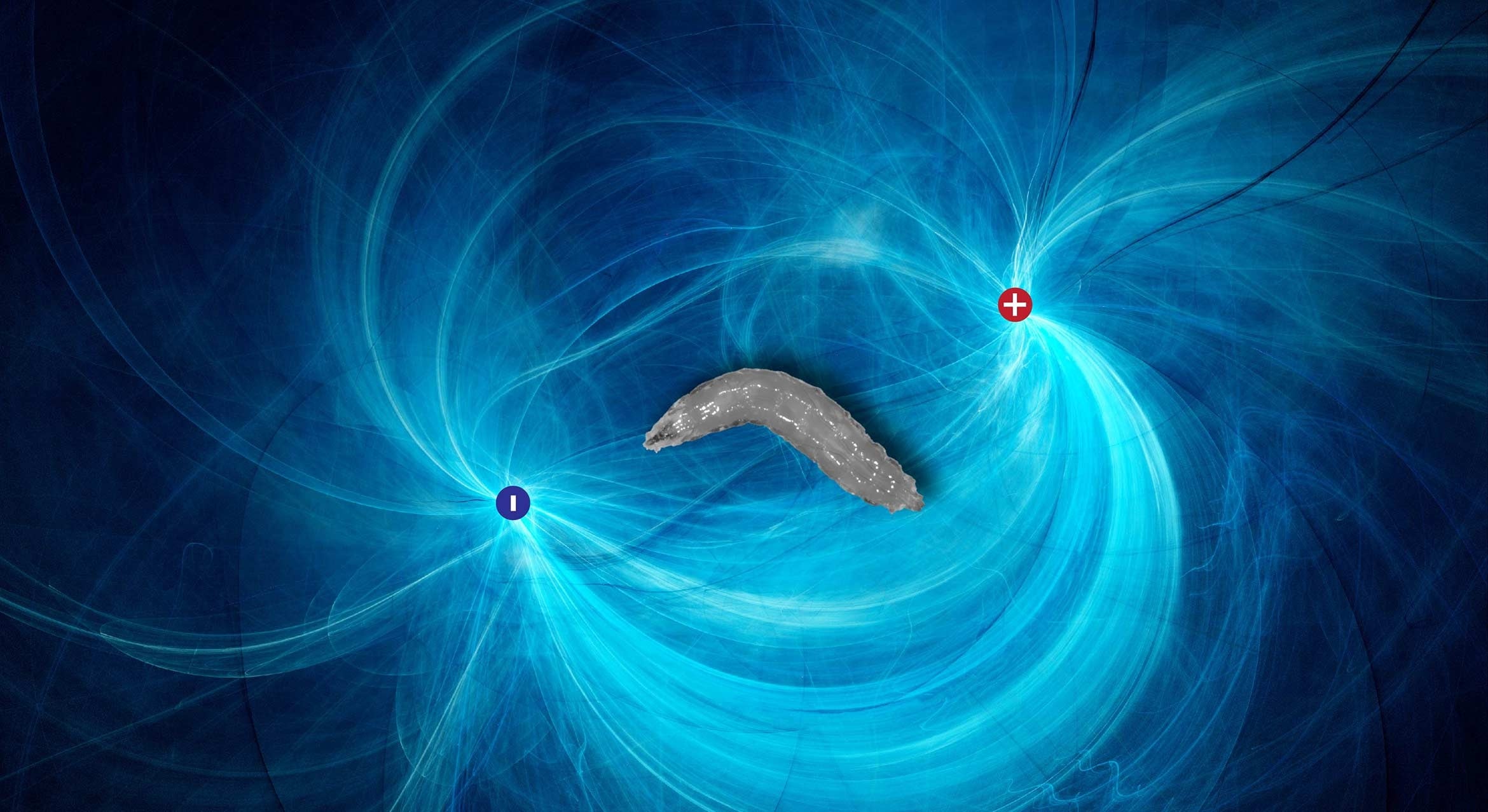
While it may be an unfamiliar sensation to humans, electroreception is relatively commonplace in the animal kingdom. Sharks, bees and even the platypus all share this ability to detect electric fields in their environment.
Scientists at UC Santa Barbara have just added fruit flies to that list. A team of researchers led by Matthieu Louis found that fruit fly larvae can sense electric fields and navigate toward the negative electric potential using a small set of sensory neurons in their head.
The findings, published in Current Biology, present an immense opportunity. Fruit flies are arguably the most commonly used experimental animals, the basis for studies in fields as disparate as genetics, neurobiology and aging. Uncovering electroreception in fruit flies opens new avenues of research into the basis of this sense and could even lead to new techniques in bioengineering.
Discovering a sixth sense in fruit flies
“To us, electro-sensation is probably something that doesn’t mean much, because we’re not responding to it. But there’s a growing recognition that, to many animals, it’s actually very important,” said Louis, an associate professor in the Department of Molecular, Cellular, and Developmental Biology.
So when he heard that the ability had been discovered in the nematode C. elegans (another commonly used model organism), he was curious to see whether the Drosophila fruit flies also had this capability.
Co-author Julia Riedl, Louis’ student at the time, set off to investigate using a routine technique in biology research: gel electrophoresis, which uses an electric field to push molecules through a gel with small pores. This is often used to sort DNA fragments, but Riedl used it to immerse a Drosophila larva in an electric field. “Everyone who runs a lab has this device,” said Riedl, who now works at Imperial College London. “Instead of putting DNA on there, we put the larvae on there, and it gave this super robust behavior.” The larva reoriented itself and began moving toward the negative electrode.
Localizing the sensation
After observing the animal’s reaction, the team wanted to locate the neurons responsible for it. For that, they needed a way to turn off different parts of the nervous system. So they targeted the gene GAL4. Promoter genes like GAL4 serve as road signs that tell the cell’s machinery where to begin copying the DNA into RNA, a crucial step in gene expression.
When activated, the GAL4 triggered the production of a modified form of tetanus toxin. This acts like a molecular “roadblock,” preventing the targeted neurons from communicating with other neurons. By using lineages of larvae with GAL4 expressed in different neurons, the team could silence specific groups of neurons to see how the animals’ behavior changed.
Silencing different neurons enabled the group to determine which ones were crucial to electroreception. They located the neurons of interest on either side of the larva’s head, near the tip, in an area involved in smell and taste sensation.
Riedl inserted a gene that codes for a protein that fluoresces when neurons are active so she could track the activity in real time. Exposing the head segment to an electric field under the microscope confirmed her initial finding. “You don’t need to do a fancy analysis,” she said. “If the neurons really respond, they light up.”
“Honestly, I couldn’t believe it. It was so clear,” she added.
In fact, only a single neuron in this cluster responded to the electric field. The neuron was inhibited when the electrode was in front of the head, and activated when the electrode was behind, triggering the larva to reorient itself.
The hard work of reducing uncertainty
Louis and Riedl began this work in 2009, when Louis was a researcher at the Centre for Genomic Regulation (CRG) in Barcelona, Spain. “It’s an interesting project because it took more than 15 years to complete,” he said. “A scientific marathon.”
Part of the reason is that electric fields are hard to measure and visualize, in contrast to magnetic fields, which can be revealed with some iron filings. The team was concerned that the larvae may be responding to other, confounding factors in the experimental setup, such as the electric current, acidity or temperature gradient.
When Louis joined the faculty at UCSB, he was able to connect with electrochemist Lior Sepunaru and mechanical engineer Alex Eden, who could model the electric field in the experiment. Eden provided the group with the sophisticated simulations they needed to characterize the environment within the experiment. And while Louis’ lab is no stranger to running simulations, “that modeling is several orders of magnitude beyond what we can typically do,” he said.
With a better account of the experimental conditions, the team could now begin systematically eliminating those confounding factors. They switched to an electrolyte solution that wouldn’t change its acidity over the course of the experiment. They also tightened their control over resistive heating in the gel.
“In order to test whether the current or the electric field was really the element driving the behavior, we wanted to change one but not the other,” Louis explained, “which is extremely difficult to achieve, because the two of them are interlocked.”
Eden’s simulations revealed that they could accomplish this by changing the thickness of the agar medium. The results confirmed their hypothesis; the larvae were responding to the electric field itself, not the current it induced in the agar gel. And it was specifically the neurons in their head that detected the electric field, its strength and orientation.
Adaptation speculation
The authors aren’t certain why Drosophila larvae evolved electroreception, but they’ve developed some hypotheses along the way. Electrosensitivity may help the larvae navigate the rotting fruit they live in. Fruit fly larvae develop in just a few days, so they need to quickly find areas with softer pulp, more sugar and less alcohol. Fermentation can create an electrical gradient that the animals then use to navigate, Louis said.
Besides, the inside of an apple is quite dark. “The senses that develop during evolution represent the physical properties of the environment,” Riedl added. “So if there is an electric field, why not sense it?”
Alternatively, it could help the animals avoid predation. Flying insects pick up a positive charge, so this aversion to positive charges may be an adaptation to help Drosophila larvae avoid parasitoid wasps, which can claim up to 90% of the larvae before they reach adulthood.
Or it could be a combination of things, “both leading them toward the more favorable part of a fruit and also leading them away from a wasp attack,” Riedl noted.
Either way, the discovery provides a better account of the Drosophila umwelt: the way in which organisms of a particular species perceive and experience the world, shaped by their sensory organs and perceptual systems.
Further questions and new opportunities
In most of the animals scientists have studied, electroreception involves a mechanosensory structure that detects movement in response to static charge, much like your arm hair twitching when you detangle fresh laundry. But the authors didn’t observe this in the Drosophila larvae: The neurons appeared to respond directly to the electric field.
There could be a hidden apparatus that the authors haven’t located yet, or there may be something very interesting happening in these electrosensory neurons.
Additionally, the neurons responsible for this sensation are embedded in a cluster that detects smells and tastes. In fact, the neurons in question still express a gene that makes them sensitive to bitter substances. It’s possible that the cells may respond to both electric fields and bitter tastes since both stimuli elicit the same response. Perhaps the positive pole of an electric field evokes a similar sensation to bitter taste in Drosophila larvae, and so they avoid them.
The group sees their discovery as a step toward understanding electroreception more broadly. “You have all these different animals that can detect electric fields. But because none of them are model organisms, it’s very hard to get at the genetic basis of the sense,” said co-author David Tadres, a doctoral student in Louis’ lab. But since Drosophila is a go-to organism for studying all sorts of genetic questions, the team is hopeful that they’ll be able to identify the genes involved in this sensation.
Studying the basis of electro-sensation has implications beyond just understanding sensory systems in animals. Cells often move in response to electric fields and currents. Research in Drosophila could shed light on this behavior, which is an important component of wound healing, Louis explained.
The discovery could also lead to novel research tools. For instance, light-responsive genes in algae led to the development of optogenetics: the ability to directly control gene expression and neural activity with light. All the molecular tools for remote controlling these activities require access to the cell, which can be quite invasive. But a technique that made use of an electric field could simply penetrate into the tissue.
“Studying a new sensory modality in a tiny Drosophila larva could open new directions" for bioengineering,” Louis said.