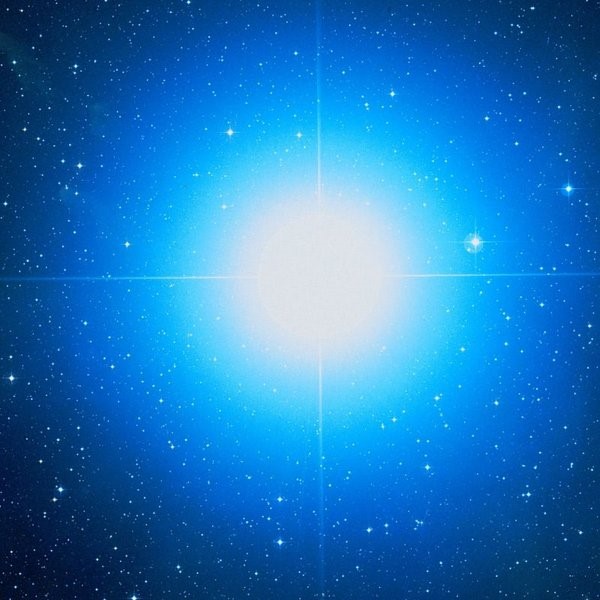
A Flash and a Shudder
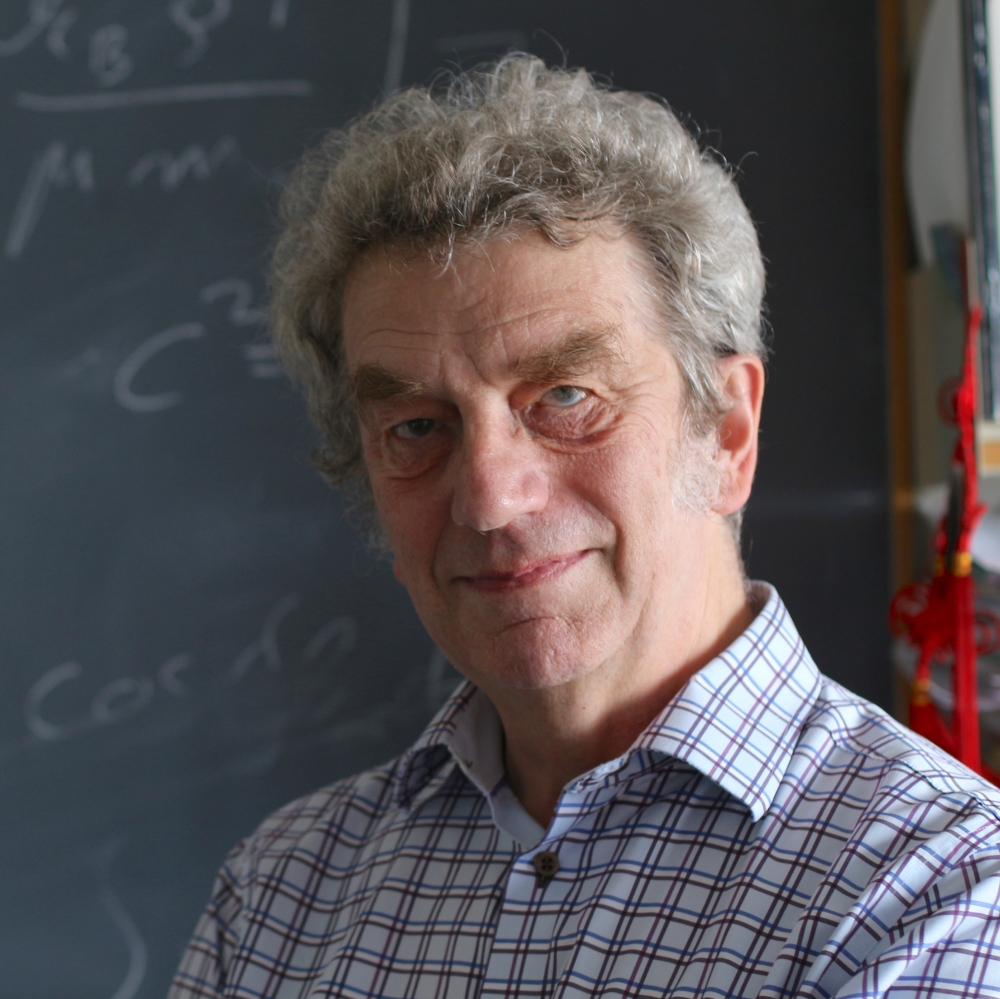
In five billion years or so, when the sun has used up the hydrogen in its core, it will inflate and turn into a red giant star. This phase of its life — and that of other stars up to twice its mass — is relatively short compared with the more than 10 billion-year life of the sun. The red giant will shine 1000 times brighter than the sun, and suddenly the helium deep in its core will begin fusing to carbon in a process called the “helium core flash.” After this, the star settles into 100 million years of quiet helium fusion.
Astrophysicists have predicted these flashes in theory and in models for 50 years, but none has ever been observed. However, a new study in Nature Astronomy Letters suggests this may soon change.
“The effects of helium core flash are clearly predicted by the models, but we have found no observations that directly reflect them,” said coauthor Jørgen Christensen-Dalsgaard, a Simons Distinguished Visiting Scholar at UC Santa Barbara’s Kavli Institute for Theoretical Physics (KITP) and professor at Aarhus University in Denmark.
A star like the sun is powered by fusing hydrogen into helium at temperatures around 15 million K. Helium, however, requires a much higher temperature than hydrogen, around 100 million K, to begin fusing into carbon, so it simply accumulates in the core while a shell of hydrogen continues to burn around it. All the while, the star expands to a size comparable to the Earth’s orbit. Eventually, the star’s core reaches the perfect conditions, triggering a violent ignition of the helium: the helium core flash. The core undergoes several flashes over the next 2 million years, and then settles into a more static state where it proceeds to burn all of the helium in the core to carbon and oxygen over the course of around 100 million years.
Helium core flash plays an integral role in our understanding of the life cycles of low-mass stars. Unfortunately, gathering data from the cores of distant stars is incredibly difficult, so scientists have been unable to observe this phenomenon.
The power of modern space-based observatories like Kepler, CoRoT and now NASA's Transiting Exoplanet Survey Satellite (TESS) promises to change this. “The availability of very sensitive measurements from space has made it possible to observe subtle oscillations in the brightness of a very large number of stars,” Christensen-Dalsgaard explained.
The helium core flash produces a series of different waves that propagate through the star. This causes the star to vibrate like a bell, which manifests as a weak variation in its overall brightness. Observations of stellar pulsations have already taught astronomers about the processes inside stars in much the way that geologists learn about the Earth’s interior by studying earthquakes. This technique, known as asteroseismology, has grown to become a flourishing field in astrophysics.
The core flash happens quite suddenly, and like an earthquake, begins with a very energetic event followed by a series of successively weaker events over the next 2 million years — a relatively short period in the life of most stars. As shown in an early paper in 2012 led by KITP Director Lars Bildsten and KITP Senior Fellow Bill Paxton, the pulsation frequencies of these stars are very sensitive to the conditions in the core. As a result, asteroseismology could provide scientists with information that tests our understanding of these processes.
“We were excited at the time that these new space capabilities might allow us to confirm this long-studied piece of stellar evolution. However, we did not consider the even more exciting possibility that these authors explored of using the vigorously convecting star to actually get the star ringing,” said Bildsten.
The main purpose of the new study was to determine whether these flashing regions could excite pulsations large enough for us to see. And after months of analysis and simulations, the researchers found that many should be relatively easy to observe.
“I was certainly surprised that the mechanism actually worked so well,” said Christensen-Dalsgaard.
The new and promising angle detailed in the paper is that the astronomers have been studying the processes in a very special — and up to now not very well understood — type of star designated a subdwarf B star. These are former red giants that, for unknown reasons, have lost most of their outer layer of hydrogen. Subdwarf B stars provide scientists a unique opportunity to more directly probe the hot core of a star. What’s more, the remaining thin layer of hydrogen is not thick enough to dampen the oscillations from the repeated helium core flashes, giving the researchers a chance to potentially observe them directly.
This study provides the first observational information about the complex processes predicted by stellar models at the ignition of helium fusion. “This work took strong advantage of a series of fluid dynamical calculations led by former KITP Graduate Fellow Daniel Lecoanet,” Bildsten noted. “If this all works out, these stars may provide a new testing ground for this fundamental puzzle in astrophysics.”
Christensen-Dalsgaard said he is eager to apply these findings to actual data. And in fact, helium core flashes may already have been observed. Several of the stars observed by CoRoT and Kepler show unexplained oscillations that appear similar to predictions of helium core flashes. TESS will prove crucial in this future research, he explained, since it will observe a whole swath of stars, including several where these pulsations may be detectable. This will provide further strong tests of the models and an insight of what the future holds for our own sun.