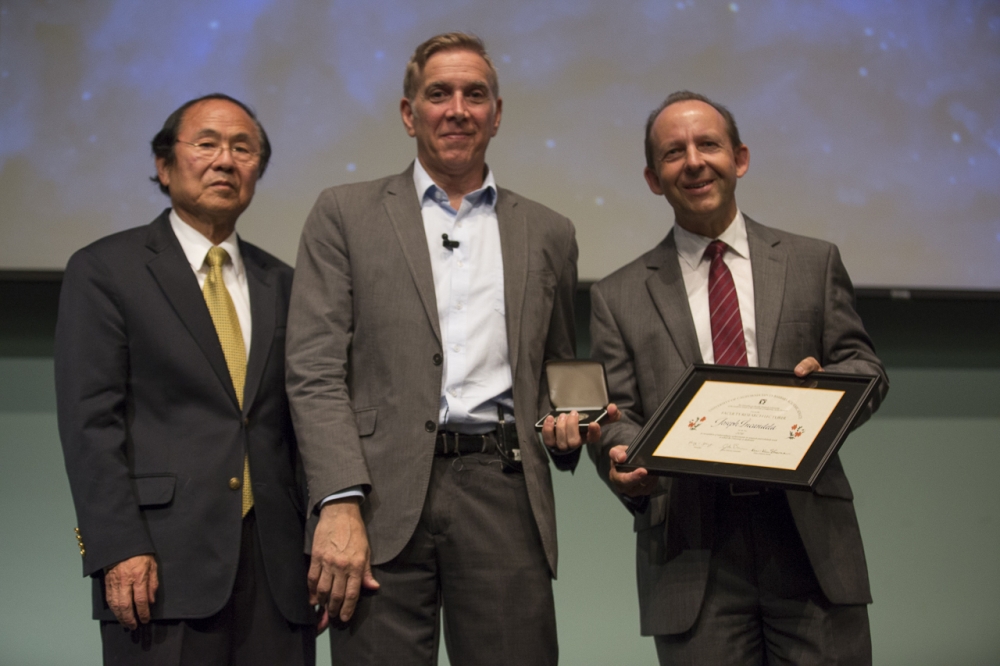
Small Particles, Enormous Machines
With humorous anecdotes, bite-sized metaphors for dense concepts in physics and the occasional pop culture reference, UC Santa Barbara physics professor Joseph Incandela gave his audience at Corwin Pavilion a rundown of research efforts to find the most fundamental units of the universe. His talk, “Searching for the Genetic Code of the Universe,” was UCSB’s 61st Annual Faculty Research Lecture.
“Peer recognition is the most valuable recognition,” said Chancellor Henry T. Yang at the start of the lecture, which is the highest honor bestowed upon UCSB faculty by their peers. “This award recognizes extraordinary scholarly distinction, high impact achievement and international recognition in one’s field.” Numerous previous recipients of the lectureship were also in attendance at the public talk.
From the early days of particle physics, when physicists such as Albert Einstein, Enrico Fermi and Satyendra Nath Bose were investigating the world of the atomic and smaller distance scales, great advances have been made, including the discovery of many of the elementary pieces of the universe. Together with brilliant theoretical ideas, what emerged from these discoveries was what is known as the Standard Model, a theory of fundamental particles and their interactions that was completed in 2012 with the discovery of the Higgs boson. Incandela, who currently holds the Joe and Pat Yzurdiaga Chair in Experimental Science at UCSB, was the leader of the Compact Muon Solenoid (CMS) experiment, one of two large, all-purpose multinational projects pursuing the Higgs at the time of the discovery.
“It means that space is not empty,” explained Incandela. Finding the Higgs proved the existence of a field that permeates the universe, slowing down otherwise massless particles from their light-speed trajectories to give them mass.
To find the smallest of particles, one needs the highest of energies. And that can only be obtained with the largest of machines, according to Incandela, an experimental physicist and member of UCSB’s High Energy Physics group.
Completing the Standard Model took a massive effort that began in the mid-1980s and resulted in the Large Hadron Collider (LHC), a 17-mile circular tunnel under France and Switzerland in which proton beams are launched at each other in an effort to observe the effects of super high-energy proton-proton collisions. It is very possibly the largest machine in the world, and part of the European Center for Nuclear Research (CERN).
“It effectively takes you back to a trillionth of a second after the Big Bang,” Incandela explained. To be able to observe the behavior of this newborn universe, a large ring full of powerful magnets and accelerating structures is needed. The proton beams cross in the middle of enormous particle detectors that register all of the information about the particles produced in these instantaneous proton-proton interactions. In the CMS experiment, where UCSB plays a large role, the trajectories of these particles are registered by a system of multiple layers of super-light silicon sensors, followed by massive systems that are used to stop the particles and measure their energies in the process. Almost all of the 80 million sensing elements in the detector are tucked inside the world’s largest superconducting magnet.
The UCSB contingent involved in the CMS experiment consists of Incandela and three other high energy physicists — Claudio Campagnari, Jeff Richman and David Stuart — eight postdocs, 14 grad students and a slew of engineers, software and information technicians and undergraduate students who help design and build parts of the detector and analyze its data.
“I’d like to thank faculty and staff across the university who supported this,” Incandela said. “Because even though we’re very far from CERN, we’ve managed to have a big impact on this program.”
The universe would have to be “impossibly” finely tuned to accept the Higgs boson — a unique and fragile particle — at the low mass at which it was discovered in 2012 if there was nothing but the Standard Model, Incandela explained. The parameters of the model would have to be tuned to 35 decimal places.
“We don’t have any examples of this kind of fine-tuning in nature,” he said. Particle physicists around the world, including many at UCSB, are now working to find evidence of Supersymmetry (SUSY), a theoretical concept that could provide an elegant solution. SUSY proposes a partner particle to each Standard Model particle to bring balance to the universe and thus eliminate any need for fine-tuning.
To find these even more elusive SUSY particles and to understand the Higgs boson in greater detail, Incandela explained, new and still-bigger machines that allow higher precision or achieve higher energies may be needed. Several have been conceptualized in China and Japan and CERN has begun studies for a much larger underground tunnel that could be used for several types of colliders.
In the meantime, efforts are underway at CERN to increase the beam intensities of the accelerator and to improve resolution and detection capabilities to handle the big increase in the number of particles that appear in the experiments each time the beams will cross. At the energy that protons are now being collided, the experiments are probing distance scales that are to an atom what an atom is to a human. Incandela discussed plans for a High Granularity Calorimeter being designed with help from UCSB physicists and engineers for use in the CMS detector starting around 2025. Physicists at UCSB and elsewhere are also on a mission to find evidence of dark matter, invisible and weakly interacting stuff that nevertheless constitutes the majority of matter in the universe, and which is predicted by SUSY.
“There’s a lot we still don’t know,” Incandela said. “We’re still looking.”
The lecture was dedicated to Joseph Incandela, Sr., and to Joe Yzurdiaga.