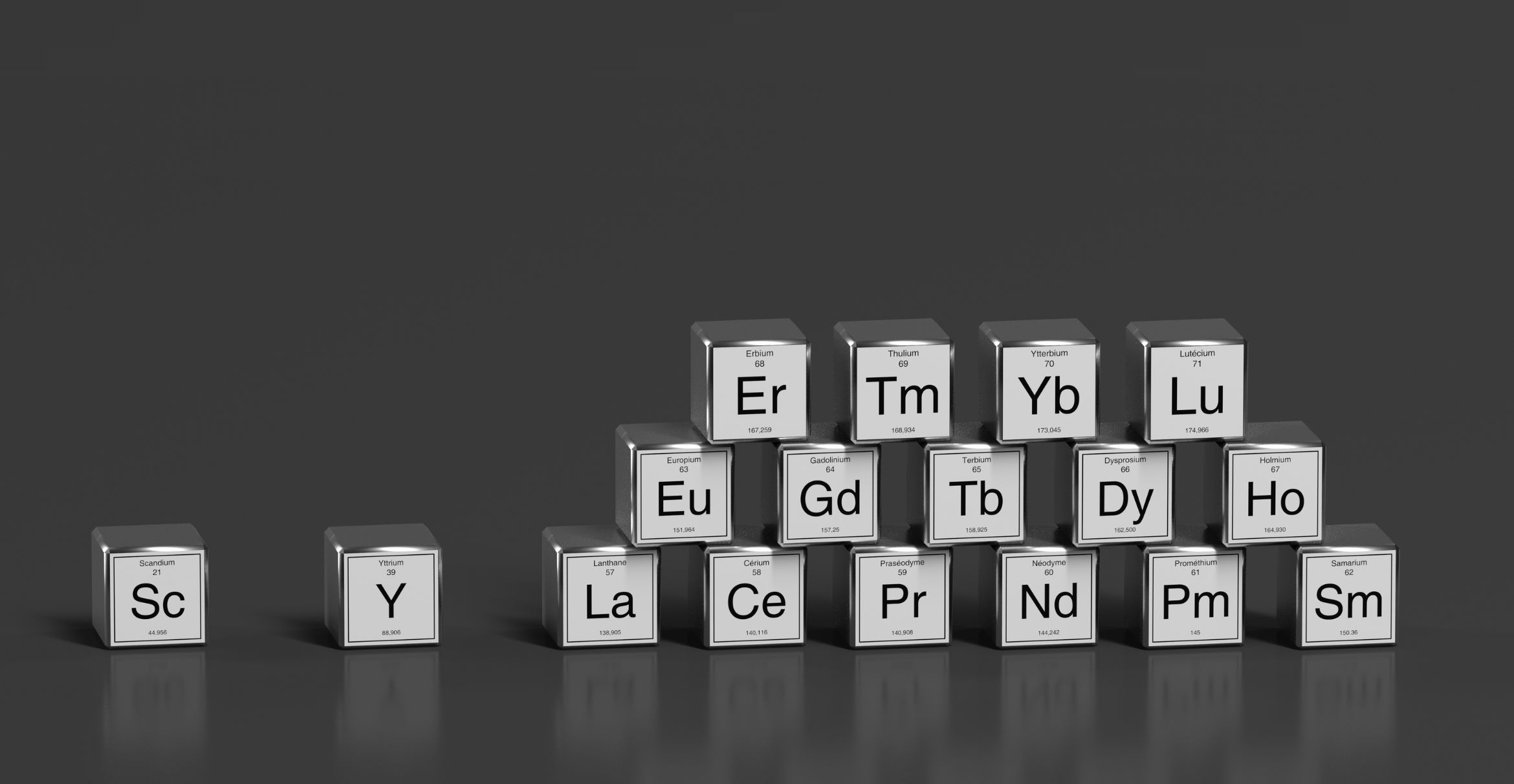
The world is going to need a lot of weird metals in the coming years, according to chemistry professor Justin Wilson at UC Santa Barbara. But he isn’t talking about lithium, cobalt or even beryllium. Wilson’s interested in dysprosium, which is so hidden in the periodic table that you’d be forgiven for thinking he made it up.
Rare earth elements (REEs) like dysprosium have a lot of niche uses in modern electronics. So much so that the U.S. Department of Energy classifies them as “critical minerals.” And while they aren’t quite as rare as noble metals like platinum or gold, they are difficult to obtain from natural deposits. They also share extremely similar chemical properties, making them devilishly difficult to isolate from one another.
But a team led by Wilson and postdoctoral researcher Yangyang Gao has just developed a technique to purify certain REEs at room temperature without relying on the toxic and caustic compounds currently used for the task. The results, published in the journal Angewandte Chemie, promise a safer, more effective way to process these metals from mining operations and recover them from electronic waste.
Useful elements out of the public eye
Rare earth elements include scandium, yttrium and the lanthanides — the first of the two rows that publishers excise from the periodic table so that it fits on a single page. The lanthanides (and actinides below them) actually slot in just to the right of the second column. You may be familiar with the REE neodymium as the metal used to make crazy strong magnets. Wilson is interested in neodymium as well.
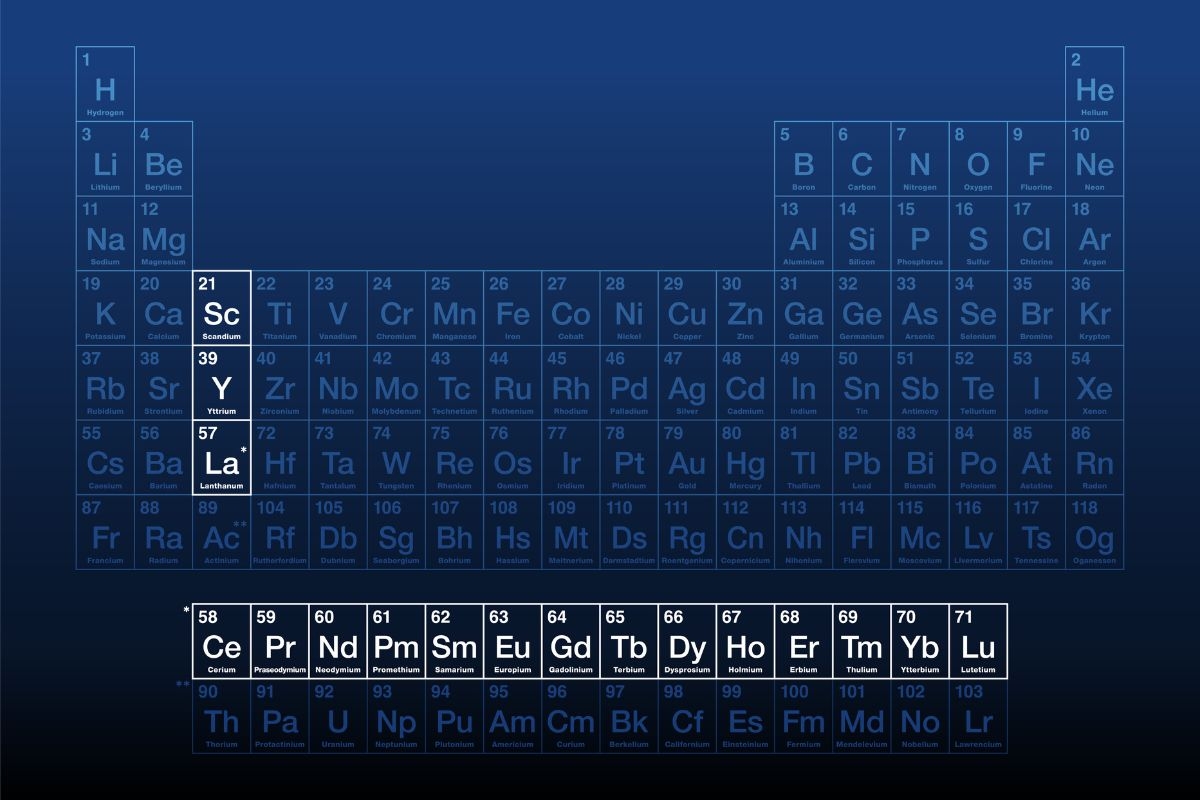
These elements share many chemical properties, making them tough to separate from one another. They all form ions with a +3 charge, and they all prefer to bond with non-metals in the second row of the periodic table (like oxygen and nitrogen). Fortunately, they differ slightly in their ionic radius, or size. However, their sizes are still fairly similar, with only a 16% change in radius across the series.
Despite their similar physical and chemical properties, the REEs do have their distinguishing features. Differences in the number and arrangement of valence electrons confer each of these elements with distinct magnetic and optical properties. Only by isolating them in pure samples can we take advantage of these unique characteristics.
Tuning the technique
The current industry standard for separating REEs from one another is called liquid-liquid extraction, which combines an organic solvent (such as a kerosene or benzene) and a water-based solvent. “It’s like salad dressing at this point, where you have two phases and they don’t mix,” Wilson said. So chemists add molecules called chelators into the organic solvent that are designed to bind to REEs.
The key is that these chelators have a slight preference for smaller atoms, which enables them to separate one type of REE from another based on size. Still the process is rather inefficient: only a couple percent enrichment for each extraction cycle. Obtaining a sufficiently pure sample of a particular element for industrial use requires many liquid-liquid extraction cycles, which generates a lot of chemical waste.
Wilson and his co-authors at Cornell and University of Nevada, Reno, developed more optimized chelators and a process that doesn’t require an organic solvent. This eliminates substances that are often inflammable, carcinogenic and toxic.
The authors tested their method in a solution of dysprosium (Dy) and neodymium (Nd). They used a specific chelator, called G-macropa, to bind to the larger Nd atoms, and then added sodium bicarbonate (aka baking soda) to cause the smaller Dy to precipitate as a carbonate salt. This can simply be filtered out and processed to recover the pure metal. Decreasing the acidity of the remaining solution enabled them to separate the Nd from the chelator, which can then be reused.
A single cycle of this new process can concentrate dysprosium by a factor of over 800, compared to less than 10 for liquid-liquid extraction.
“[I was very surprised] when my postdoc, Yangyang, showed me the elemental analysis data,” Wilson said. After repeating the test to confirm the results, the team realized just how fine-tuned their chelator was for this separation process.
By collaborating with David Cantu, a professor at UN Reno, they were able to understand and compare the efficacy of G-macropa to other chelators at a molecular level. These theoretical studies will help scientists design second-generation analogues.
Large applications and small adjustments
This efficiency is important for scaling the process because the G-macropa chelator is more complex, and thus expensive, than the ones in standard use. The team is also exploring chelators that may be less expensive to produce.
Wilson and his co-authors focused on separating Nd from Dy because the two elements are abundant in e-waste, particularly those neodymium magnets. Indeed, they performed their experiments on e-waste to emphasize its potential for making recycling an economically viable source of REEs.
They’re working to tailor this technique to other assemblages of rare earth elements as well as ensuring it works with high concentrations of REEs more similar to industrial sources.
Advances in separating rare earth elements could massively impact the supply chain for these metals. The United States has large deposits of REEs, but important environmental and health regulations have prevented American industries from competing with China, where these safeguards are much more lax.
“A cleaner and more efficient separation of these elements could potentially open up domestic supplies of the rare earth elements,” Wilson said. This would be a win-win for national security and the American economy as these weird metals become ever more important.