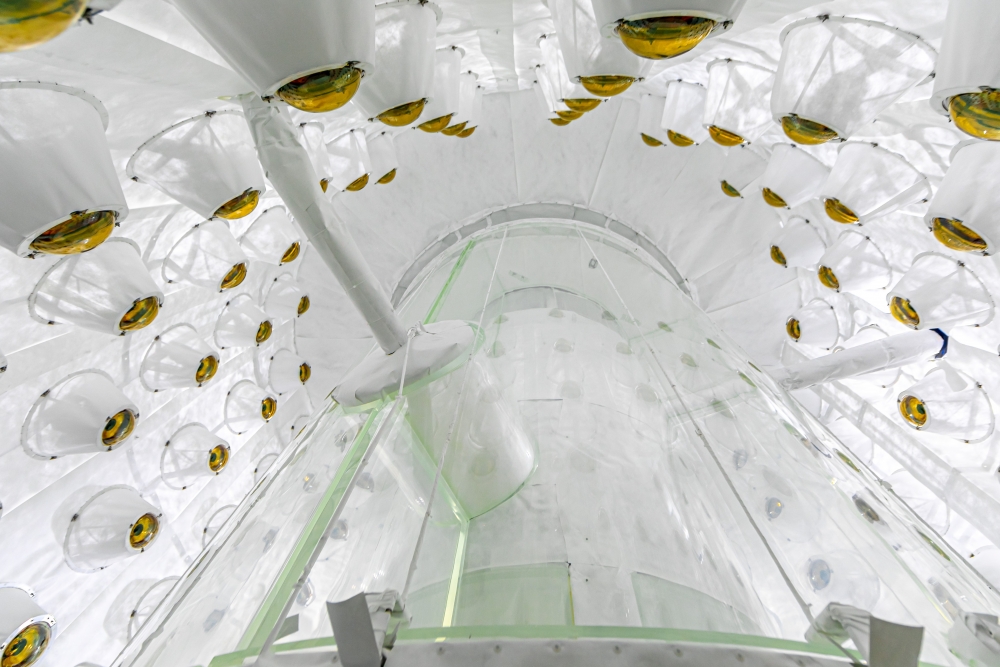
‘Out of the Starting Gate’
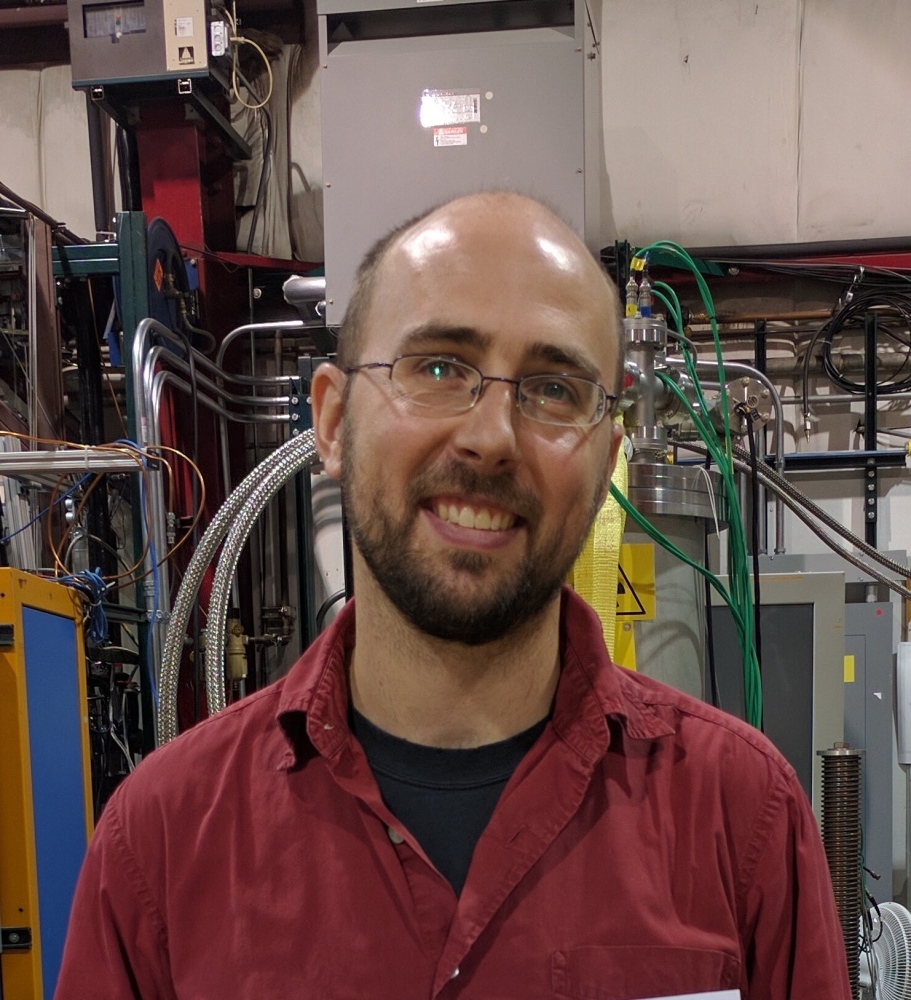
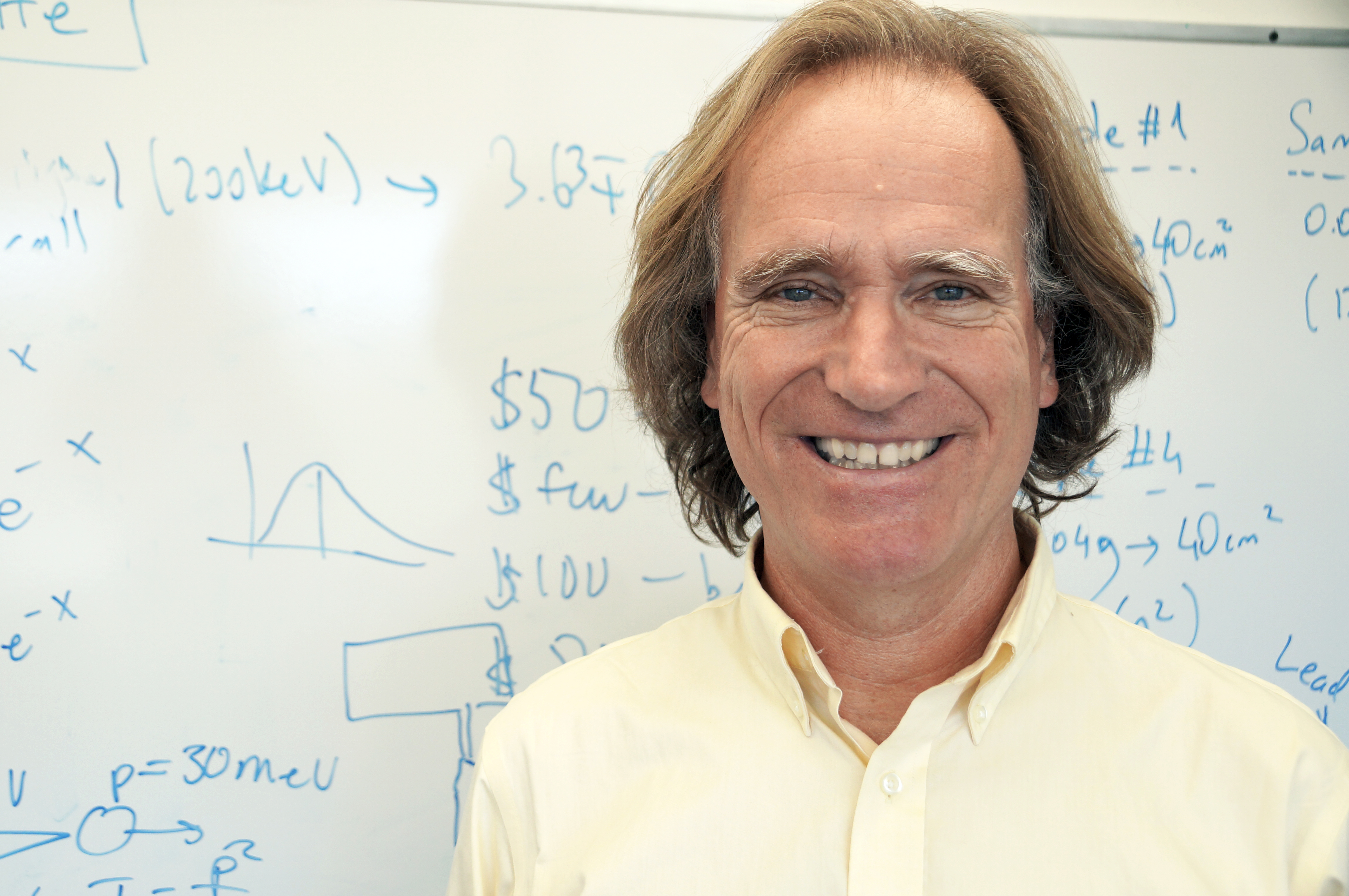
Deep below the Black Hills of South Dakota in the Sanford Underground Research Facility (SURF), sits an innovative and uniquely sensitive dark matter detector. The LUX-ZEPLIN (LZ) experiment, as it is called, has passed a check-out phase of startup operations and delivered first results.
“We are now out of the starting gate,” said Harry Nelson, a UC Santa Barbara professor of physics and spokesperson of LZ from 2014-2017. “LZ is a far more powerful detector of dark matter than any ever built before, and is uniquely capable of making a discovery in the next few years.”
In a paper posted on the experiment’s website, researchers report that with the initial run, LZ is already the world’s most sensitive dark matter detector. And, added current LZ Spokesperson Hugh Lippincott, also of UCSB, “We plan to collect about 20 times more data in the coming years, so we’re only getting started. There’s a lot of science to do, and it’s very exciting!”
Dark matter particles have never actually been detected — but perhaps not for much longer. The countdown to convincing laboratory detection of dark matter may have started with results from LZ’s first 60 “live days” of operation. These data were collected over a three-and-a-half-month span of initial operations beginning in late December 2021. This was a period long enough to confirm that the detector was functioning well.
Unseen, because it does not emit, absorb or scatter light, dark matter’s presence and gravitational pull are nonetheless fundamental to our understanding of the universe. Estimated to be about 85% of the total mass of the universe, dark matter shapes the form and movement of galaxies and is invoked by researchers to explain what is known about the large-scale structure and expansion of the universe.
The heart of the LZ dark matter detector consists of two nested titanium tanks filled with ten tonnes of very pure liquid xenon, viewed by two arrays of photomultiplier tubes able to detect faint sources of light. This “heart” is part of a larger system; a xenon “skin” layer exists immediately outside the central detector, and the titanium tanks are surrounded by a much larger Outer Detector, or “OD,” consisting of acrylic tanks filled with gadolinium-loaded liquid scintillator. The skin and the OD catch particles that might mimic a dark matter signal.
The design, manufacturing and installation phases of the LZ detector were led by Gil Gilchriese of Lawrence Berkeley Lab (LBL) in conjunction with an international team of 250 scientists and engineers from 35 institutions from the U.S., U.K., Portugal, and South Korea. LBL continues to lead the day-to-day operations of the LZ facility. LBL continues to lead the day-to-day operations of the LZ facility.
Together, the collaboration is hoping to use the instrument to record the first direct evidence of dark matter, the so-called missing mass of the cosmos.
Postdoctoral researcher Sally Shaw with one of the Outer Detector's acrylic tanks
Photo Credit: HARRY NELSON
UC Santa Barbara postdoctoral researcher Sally Shaw was the data analysis and deputy physics coordinator for this analysis. “This first result represents an unprecedented effort from an international team of all levels: undergrads, grad students, postdocs, professors, engineers and interns,” she said. “So many people have given so much to the experiment to get us to this stage, and I’m looking forward to seeing what else LZ can do.”
An underground detector
Tucked away about a mile underground at SURF in Lead, South Dakota, LZ is designed to capture dark matter in the form of weakly interacting massive particles (WIMPs). The experiment is underground to protect it from cosmic radiation at the surface that could drown out dark matter signals.
Particle collisions in the xenon produce visible scintillation, or flashes of light, that are recorded by the photomultiplier tubes, explained Berkeley Lab’s Aaron Manalaysay, who, as physics coordinator, led the efforts to produce these first physics results. “The collaboration worked well together to calibrate and to understand the detector response,” he said. “Considering we just turned it on a few months ago, and during COVID restrictions, it is impressive we have such significant results already.”
The collisions also will knock electrons off xenon atoms, sending them to drift to the top of the chamber under an applied electric field. At the top, they produce another flash, permitting spatial event reconstruction. The characteristics of the scintillation help determine the types of particles interacting in the xenon.
Physicists Nelson and Michael Witherell (formerly of UCSB, now director of LBL), led a team at UCSB that shepherded all aspects of the design. This included commissioning the Outer Detector, consisting of four 12-foot-tall, clear acrylic tanks that surround the core detector, plus five more tanks above and below. The OD is critical for a discovery, rather than a highly sensitive "null" search of dark matter interactions because a well-known particle, the neutron, can also produce a signal in liquid xenon that only the OD can distinguish.
The OD, with a budget of $5 million, was engineered in the UCSB physics department by a team including Dean White (now retired), Susanne Kyre and Dano Pagenkopf. UCSB had many partners in OD fabrication and installation, including Reynolds Polymer Technology in Grand Junction, Colo.; the Brookhaven National Lab in New York; the Korean Center for Underground Physics; Brandeis University; and the University of Liverpool.
Lippincott managed the design and installation of the xenon skin system, as well as a key component of the xenon handling system: the Xenon Tower. The xenon is continuously circulated as a gas through a purification system, turning over once every three days or so; the Xenon Tower is at heart a highly efficient heat exchanger that can provide cooling to liquid xenon returning to the detector.
UCSB postdoctoral researcher Alissa Monte was the data quality coordinator for this run. “I think the most exciting thing about this result is actually seeing what we can do next,” she said. “This result was all about demonstrating that LZ works, and it does! As we take more data and mature our analyses, we get to make LZ sing. In particular, LZ has the strongest veto systems of any large xenon experiment, and there is a lot of room to explore what physics can be done with such excellent detectors — OD, skin and time projection chamber — working in tandem.”
“I'd like to express gratitude to the large number of people who provided remote support throughout the construction, commissioning and operations of LZ, many of whom worked full time from their home institutions making sure the experiment would be a success and continue to do so now,” said Tomasz Biesiadzinski of the Stanford National Accelerator Laboratory, the LZ detector operations manager. One of those people was UCSB postdoctoral researcher TJ Whitis, who spent many months on site as xenon handling coordinator, leading the critical step of filling the detector with xenon, alongside UCSB graduate student Jack Bargemann. Nelson also spent many months on site on the OD installation, and monitoring the OD full time for the past year.
The LZ effort at UCSB is supported by the U.S. Department of Energy, Office of Science, Office of High Energy Physics, and by many efforts of the UCSB Physics Department, the College of Letters and Science Division of Mathematical, Life, and Physical Sciences, and the UCSB Office of Research.