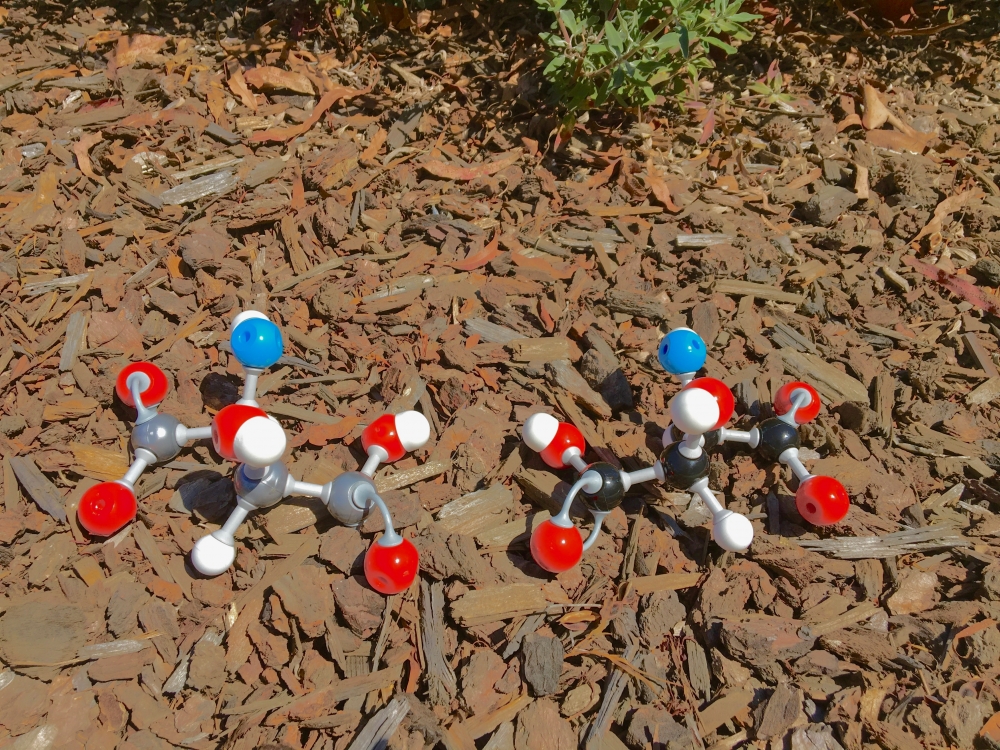
Seeing in Stereo
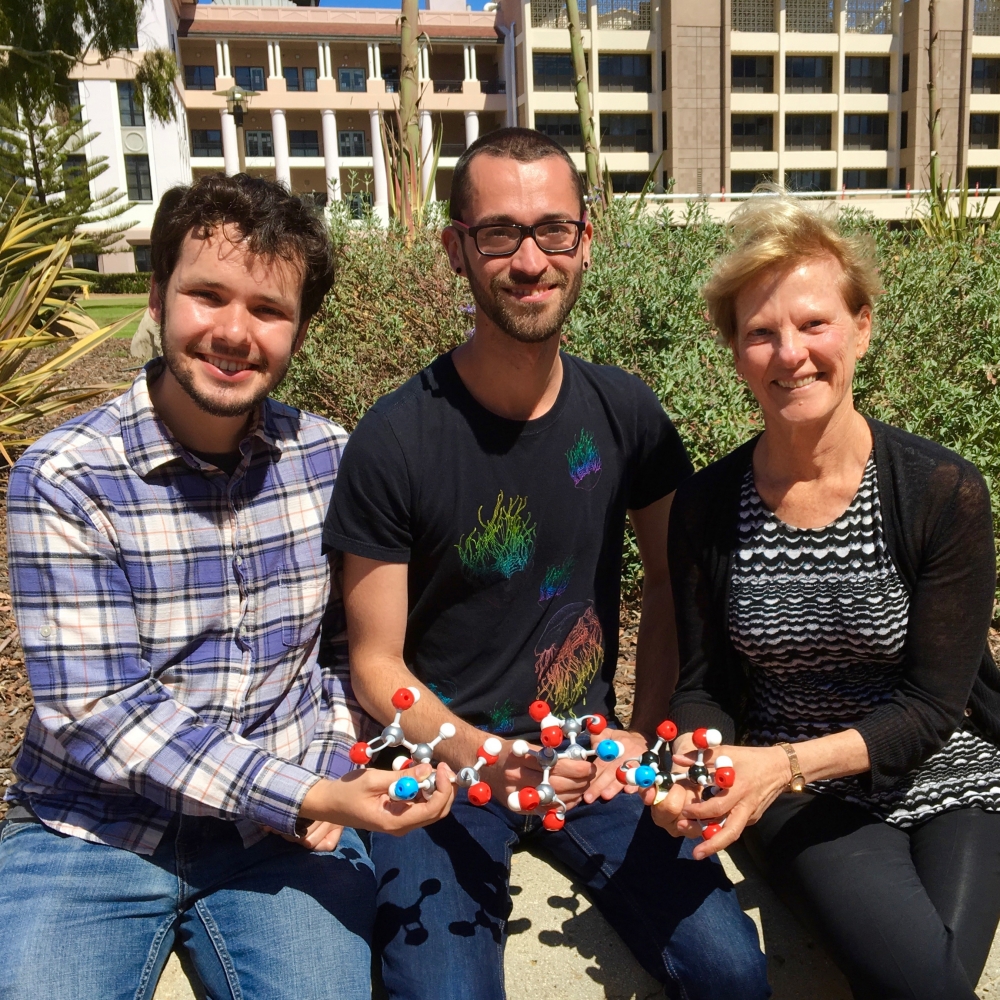
Knowing a compound’s composition isn’t always enough to understand its chemistry — sometimes you need to know how its atoms are arranged in three-dimensional space, its stereochemistry, as well.
This was precisely the case with siderophores, important bio-molecules that bind and transport iron in microorganisms. Scientists could decode their chemical composition from bacterial genomes, but that didn’t provide a complete picture of the molecule itself.
Now a group that includes UC Santa Barbara graduate students Zach Reitz and Cliff Hardy has discovered a way to decipher the 3D structure of these compounds directly from the genes that code for them. The results have ramifications for our understanding of microbial evolution and ecology and may find use in treating infections. The discovery appears in the Proceedings of the National Academy of Sciences.
“We’re interested in predicting from the genome sequence what these molecules are, including the stereochemistry,” said Reitz and Hardy’s advisor Professor Alison Butler, a distinguished professor in UC Santa Barbara’s Department of Chemistry & Biochemistry.
Butler and her lab study siderophores, small iron-binding molecules made mostly by bacteria. Life needs iron to function, but bioavailable iron is often scarce in the environment. To get around this, bacteria produce siderophores, which they send out to source iron. “Then the bacteria can bring the siderophore-iron complex back into the cell, where the iron can be used for cellular processes,” explained Hardy.
While the topic is intriguing on a fundamental level, some of the bacteria that use siderophores are human pathogens. A better understanding of these compounds could help us thwart bacterial growth and treat illness.
Much like proteins, siderophores often consist of several amino acids linked together in a chain, a class of molecules scientists call a peptide. However, they’re smaller than proteins and often include amino acids that are not one of the 20 found in proteins. Scientists can fairly easily predict a siderophore’s composition from the genes that code for the enzymes that produce it. This leaves open the nature of the compound’s stereochemistry at the iron-binding sites, a question that had been vexing scientists for decades.
With this in mind, Reitz began organizing a family tree for a particular class of enzymes that perform a key step in the assembly of siderophores. Four subfamilies of these enzymes emerged, which clustered into two major groups. “And strangely, sometimes a genome would code for both [groups],” said Reitz. “It seemed redundant, at first, that it would have both of them.” So, they began looking for patterns to account for this peculiarity.
The researchers were surprised to find that the enzymes gave rise to siderophores with structures that followed the distinctions between the subfamilies. Notably, the major group that the enzyme belonged to directly impacted stereochemistry of the siderophore it produced.
“Until we did this work, you couldn’t just look at the genome and predict the stereochemistry,” said Hardy. The technique proved so reliable that the team was even able to correct a mistake they found in the literature, where the genome prediction didn’t match the described structure. “It turned out that the prediction was right and the published stereochemistry was wrong,” said Hardy.
“The genome is a strong guide to the structure,” added Butler. “So if you get chemistry that doesn’t agree with the genome, go back and check the chemistry.”
Now the group wants to determine whether different stereochemistries affect the biology of iron uptake. “That’s what we’re working on now, trying to figure out the implications of the stereochemistry on the iron chemistry,” said Hardy. They’re also interested in how these differences evolved, which came first and what was the first siderophore.
“Bacteria are better chemists than we will ever be,” said Reitz. “An organic chemist would be ecstatic if they could get this stereo-selectivity in a lab as well as the bacteria can do it.”
“Controlling stereochemistry might be the most difficult thing to do in synthetic chemistry,” added Hardy, “and these bacteria just do it without an issue.”