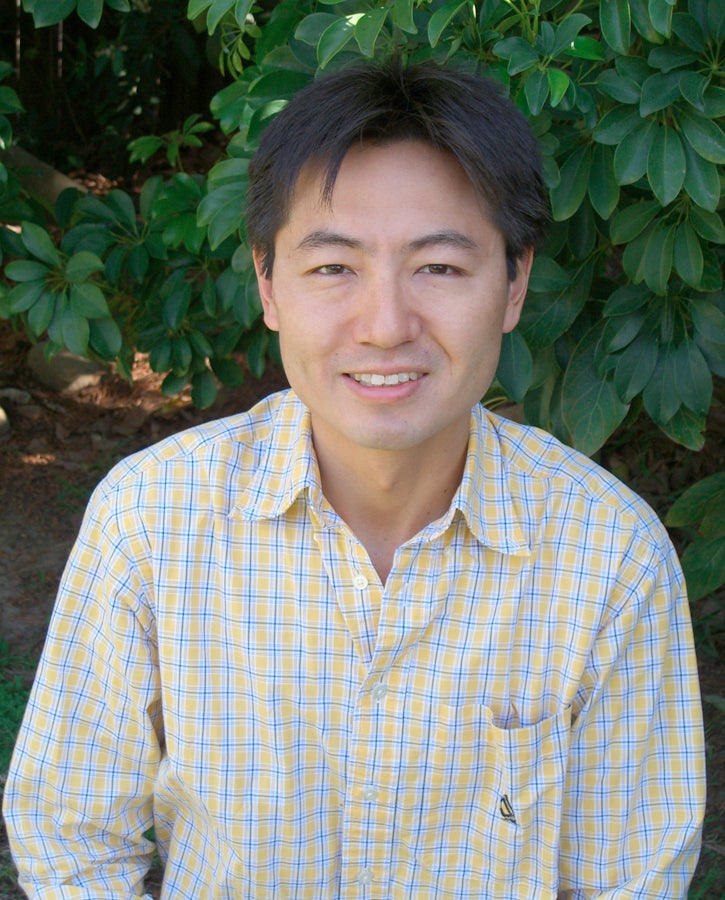
Scientists have taken a new step toward quantum computing, which will use quantum mechanics to revolutionize the way information is processed. This research appears today in the online version of Nature magazine.
First author Susumu Takahashi conducted the research as a postdoctoral researcher at UC Santa Barbara's Institute of Terahertz Science and Technology and the Department of Physics. Takashi's supervisor at UCSB was Mark Sherwin, professor of physics and director of the Institute of Terahertz Science and Technology. Takahashi is now an assistant professor in the University of Southern California's Dornsife College of Letters.
Quantum computers will capitalize on the mind-bending properties of quantum particles to perform complex calculations that are impossible for today's traditional computers.
Using high magnetic fields, Takahashi and his colleagues managed to suppress decoherence, which is one of the key stumbling blocks in quantum computing.
"High magnetic fields reduce the level of the noises in the surroundings, so they can constrain the decoherence very efficiently," Takahashi said. Decoherence has been described as a "quantum bug" that destroys fundamental properties that quantum computers would rely on.
Quantum computing uses quantum bits, or qubits, to encode information in the form of ones and zeros. Unlike a traditional computer that uses traditional bits, a quantum computer takes advantage of the seemingly impossible fact that qubits can exist in multiple states at the same time, which is called "superposition."
While a bit can represent either a one or a zero, a qubit can represent a one and a zero at the same time due to superposition. This allows for simultaneous processing of calculations in a truly parallel system, skyrocketing computing ability.
Though the concepts underpinning quantum computing are not new, problems such as decoherence have hindered the construction of a fully functioning quantum computer.
Think of decoherence as a form of noise or interference, knocking a quantum particle out of superposition –– robbing it of that special property that makes it so useful. If a quantum computer relies on a quantum particle's ability to be both here and there, then decoherence is the frustrating phenomenon that causes a quantum particle to be either here or there.
Co-authors at the University of British Columbia (UBC) calculated all sources of decoherence in their experiment as a function of temperature, magnetic field, and by nuclear isotopic concentrations, and suggested the optimum condition to operate qubits, reducing decoherence by approximately 1,000 times.
Qubits in this experiment were predicted to last about 500 microseconds at the optimum condition.
Decoherence in qubit systems falls into two general categories. One is an intrinsic decoherence caused by constituents in the qubit system, and the other is an extrinsic decoherence caused by imperfections in the system, for example, impurities and defects.
In their study, Takahashi and his colleagues investigated single crystals of molecular magnets. Because of their purity, molecular magnets eliminate the extrinsic decoherence, allowing researchers to calculate intrinsic decoherence precisely.
"For the first time, we've been able to predict and control all the environmental decoherence mechanisms in a very complex system –– in this case, a large magnetic molecule," said Phil Stamp, UBC professor of physics and astronomy and director of the Pacific Institute of Theoretical Physics.
Using crystalline molecular magnets allowed researchers to build qubits out of an immense quantity of quantum particles, rather than a single quantum object –– the way most proto-quantum computers are built at the moment.
"This will obviously increase signals from the qubit drastically, so the detection of the qubit in the molecular magnets is much easier," Takahashi said.
Research for the article was performed in collaboration with Stamp and Igor Tupitsyn of UBC, Johan van Tol of Florida State University, and Chris Beedle and David Hendrickson of UC San Diego.
This work was supported by the National Science Foundation; the W. M. Keck Foundation; the Pacific Institute of Theoretical Physics at UBC; the Natural Sciences and Engineering Research Council of Canada; the Canadian Institute for Advanced Research; and the USC startup funds.